SKELETAL MUSCLE PHYSIOLOGY
NEUROMUSCULAR JUNCTION
An important part of understanding the full story of muscle contraction is understanding how a nerve communicates an electrical signal to a muscle fiber. To help with this, you should complete the following steps before reading further.
Step 01
Download a page sized image of the neuromuscular junction.
Step 02
Complete an image labeling tutorial and label your own blank image.
Step 03
With your newly labeled image in hand, finish the rest of the reading on this page.
To understand how skeletal muscle fibers contract, we need to appreciate the relationship between the electrical event, or excitation, and the mechanical event, or contraction. Let's discuss a true story experienced by Dr. Davis while practicing as a physician.
Story Time
Dr Davis got a call from the emergency department (ED) at the hospital in Idaho Falls, asking him to come in and see a patient with holes in both ear drums. Perforated tympanic membranes aren't usually a serious emergency, but when the ED doctor told Dr. Davis that the patient had been struck by lightning, he headed right in to the hospital. What had occurred is that the patient was a fly-fishing guide on the South Fork of the Snake River. His clients for the day were a married couple from the east coast. They noticed some lightning and storm clouds in the distance, but weren't too worried. However, a minute or two later there was an ear-shattering crash, and the guide felt like he was grabbed by an immense hand, lifted out of the boat, violently shaken, then slammed back into the boat. When he managed to recover a bit, the husband was looking ghostly white and shaken. Part of the shock was that the wife was just gone. The husband told the guide that the boat had been struck by lightning, and his wife had disappeared. They figured she was probably dead, but began looking around the boat and fortunately saw her body floating downstream. They hurriedly rowed over to her and pulled her into the boat. Happily, the movement seemed to revive her, and she started breathing again and opened her rather dazed eyes. When the boat was struck by lightning, the current had passed through the graphite oars into the surrounding water. Unfortunately, the guide had his hands on the oars when they decided to become lighting rods, so he got hit with a lot of the current. They weren't quite sure why the wife had been blown out of the boat, but fortunately were able to save her.
The reason Dr. Davis was called, is that both of the guide's ear drums had been vaporized. He noticed that his hearing was really bad. For some reason they decided to quit fishing (which is generally a bad idea, but reasonable in this case), pulled off the river, and called 911. Everybody eventually ended up being fine, but the reason the story is mentioned is to explain the difference between the electrical event (the lightning strike) and the mechanical event (the vanishing ear drums, shaken-up guide, and disappearing wife). Even though their misfortune helps us learn some really important muscle physiology, I want to reemphasize the tragic nature of this event. Up until that point had been a really great day, but a pesky minor problem like a lightning strike made them miss hours of fishing.
Sliding Filament Model of Muscle Contraction
A similar, although less dramatic, connection occurs in skeletal muscle physiology. In order for these muscle fibers to contract, there needs to be an electrical event-an action potential that is followed by a mechanical event-the contraction of the muscle fiber. Because you have already learned about the resting membrane potential and the action potential, we'll move past that and talk about the sliding filament model of muscle contraction.
Recall that we have already mentioned the fact that the thick and thin myofilaments slide over each other, like the parts of an extension ladder. The proteins themselves don't shorten. The muscle contraction and shortening occurs as the myofilaments grip each other, slide past each other, and shorten the sarcomeres. Thus, this is known as the sliding filament model of muscle contraction. Let's also remember that in order for action potentials to both start and propagate (travel), it is necessary for various ion channels to open and close at just the right time. Some of these ion channels open in response to binding of a ligan-an atom or molecule that binds to a receptor and stimulates a specific response. These types of ion channels are known as ligand-gated ion channels. Other ion channels open in response to a change in voltage-electricity, and are known as voltage-gated ion channels. Now we'll discuss the sequence of events that occur when and action potential reaches the end of the motor neuron.
- An action potential arrives at the axon terminal of a somatic motor neuron. Remember that in skeletal muscle, no stimulation by a motor neuron means no contraction. (This is not necessarily true for smooth and cardiac muscle, but we'll get to that later.) The axon terminal of the motor neuron connects to the muscle fiber via the neuromuscular junction (a synapse).
- The arrival of the action potential stimulates Voltage-gated Ca2+ channels in the axon's membrane to open, and Ca2+ enters the axon terminal from the extracellular space.
- The axon terminal contains synaptic vesicles filled with the neurotransmitter acetylcholine (ACh). The increased Ca2+ levels is the signal that stimulates exocytosis of these synaptic vesicles and the release of ACh into the synaptic cleft.
- The ACh diffuses across the synaptic cleft, binding to acetylcholine receptors on the ligand-gated ion channels (nicotinic type I) in the sarcolemma of the post synaptic tissue, the muscle fiber. This specialized region of the sarcolemma is known as the motor end plate, and this is the location of the ACh receptors.
- ACh binding causes ligand-gated Na+/K+ channels to open. These ion channels are permeable to both Na+ and K+ , however, more Na+ diffuses into the cell than K+ diffuses out of the cell. This may seem odd since both would be moving down their concentration gradients. The difference has to do with the charge on the membrane. Since the inside of the cell is negative, which will attract Na+ , Na+ will be moving down both its concentration and its electrical gradients. Potassium, on the other hand, would move down its concentration gradient but against its electrical gradient (the negative charge inside the cell will attract the K+ . The Na+ entering the cell depolarizes the sarcolemma which then will cause the voltage-gated Na+ channels to open, initiating an action potential that spreads out from the neuromuscular junction. The action potential not only travels across the sarcolemma, but also down the T tubules. Remember, T tubules are just invaginations (inward protrusions) of the sarcolemma, and so are filled with extracellular fluid-high in sodium (Na+) and low in potassium (K+). Also please notice that the ACh receptors are ligand-gated, but movement of Na+ through them causes voltage gated Na+ channels to open, resulting in generation and propagation of an action potential.
- While the action potential spreads, let's take a break and describe how the stimulation of the ACh receptors is terminated. For the muscle to relax ACh must be removed from the synaptic cleft. This is done when ACh is cleaved (split) by an enzyme that resides in the cleft called acetylcholinesterase. This enzyme splits ACH into its two components, acetate (acetyl) and choline, rendering it nonfunctional. The acetate portion of acetylcholine diffuses out of the synaptic cleft. The choline, which is an essential nutrient in the Vitamin B group ( B 4 ), is taken up by the axon terminal, where it is recycled to make more acetylcholine. Although our bodies can make choline, we cannot produce enough for our needs and must get it in our diet and recycle what we have.
- The action potential does its thing (If you have forgotten the basics of action potentials review module 5).
- As the action potential spreads along the sarcolemma and the T-tubules, the resultant change in potential causes other voltage gated channels in the T-tubule to respond. These channels are called dihydropyridine channels (DHP) or L-type Ca2+ channels, and are mechanically linked to ryanodine receptor channels (RyR) , calcium channels located in the sarcoplamsic reticulum membrane. These two protein channels span the distance between the T-tubule and the terminal cisternae of the sarcoplasmic reticulum. In response to the change in membrane potential, the DHP channel causes the RyR to open and allows Ca2+ ions to leave the sarcoplasmic reticulum and diffuse into the sarcoplasm. These calcium ions bind to troponin causing it to move the tropomyosin molecules off of the active sites on each G-actin molecule.
- Uncovering the active sites allows the mysoin heads to bind to actin binding sites, forming cross bridges. In the resting state, the myosin head is "cocked" and ready to go. It also has ADP and phosphate (Pi) attached to it. The binding to actin causes Pi to detach from the myosin head resulting in the release of energy which causes the myosin head to bend (see the image below). This bending or power stroke forcefully pulls the actin past the myosin. During the power stroke the ADP is also released from they myosin. Recall the arrangement of the thick filaments with half of the myosin molecules pointing one way and half pointing the other. Since the myosin heads on the opposite ends of the thick filaments all pull towards the middle, the overall effect is to cause the sarcomere to shorten. As all of the sarcomeres in the muscle fiber shorten, the entire muscle shortens or contracts.
- In order for significant shortening of a skeletal muscle fiber to occur, the myosin heads must now detach from the G-actin active sites and then re-attach to a different active site further along the neighboring actin molecule. This is rather like the fact that in order to climb a ladder we must pull ourselves up a rung, and then let go and move our hands and feet to higher rungs. In order for this release to occur, each myosin head must bind an ATP molecule. The binding of ATP to the myosin head allows it to release from the actin. The ATPase then hydrolyzes the ATP into ADP and a phosphate group which causes the head to "recock" (the recovery stroke),preparing it for the next power stroke. Hence, binding ATP allows the head to release and hydrolysis of ATP re-energizes the head for the next power stroke. During a single muscle cell contraction, each myosin molecule undergoes the entire cross-bridge cycle many times-a process known as cross-bridge cycling. As long as Ca2+ is present and the active sites are exposed, the process will continue.
One other important concept: Using the analogy above, when we climb a ladder, we don't take both hands off of the rungs at the same time. Likewise, when muscles contract, the myosin heads are cycling asynchronously, meaning that they don't all bind actin at the same time, and they don't all release at the same time. At any given time, the 300 or so myosin heads in one thick filament will be at different stages of the cross-bridge cycle.
The movement of myosin heads occurs in two phases:
- The power stroke or working stroke occurs when the myosin heads bend and ratchet the actin molecules past the myosin, and
- The recovery stroke involves the myosin heads detaching from actin and being cocked back into the high energy position to prepare for the next power stroke.
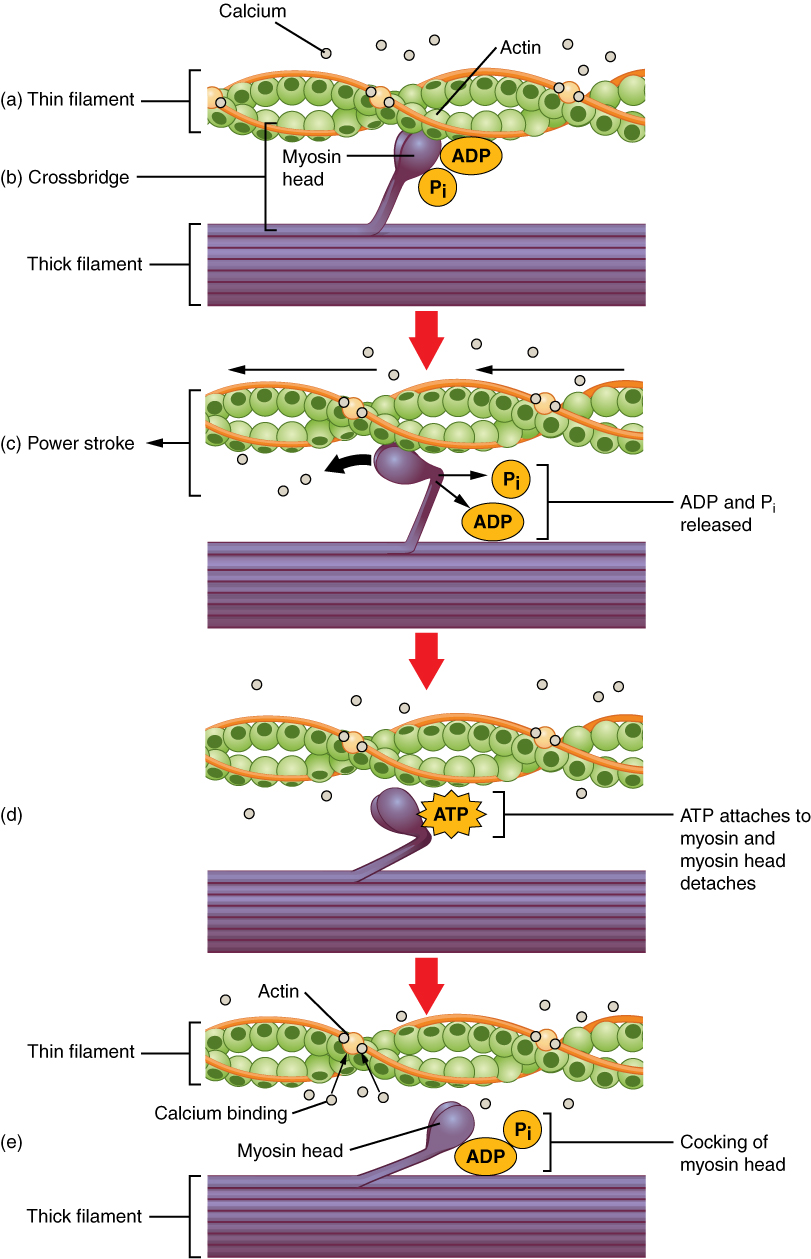
Downloaded from Wikimedia Commons Dec 2013; Author: OpenStax College; Source: ; License: Creative Commons Attribution 3.0 Unported license.
Relaxation occurs when the release of acetylcholine ceases at the neuromuscular junction. The acetylcholine already in the synapse is broken down by acetylcholinesterase, ending action potential generation and propagation. This stops Ca2+ release from the sarcoplasmic reticulum (SR). Ca2+ ions diffuse away from troponin as the Ca2+ is actively transported back into the SR. This allows the troponin-tropomyosin complex to resume its resting position, blocking the active binding sites on the individual G-actin molecules. This prevents cross bridges from reforming and results in muscle relaxation.
To quickly review: the sliding filament model of muscle contraction explains the fact that when skeletal muscle fibers contract, the individual proteins (actin and myosin) don't shorten. Rather, they slide over each other. ATP is necessary for detachment of myosin heads from actin. Notice also that when a sarcomere contracts, both the H zone and the light I band shrink in width, while the dark A band doesn't appear to narrow.
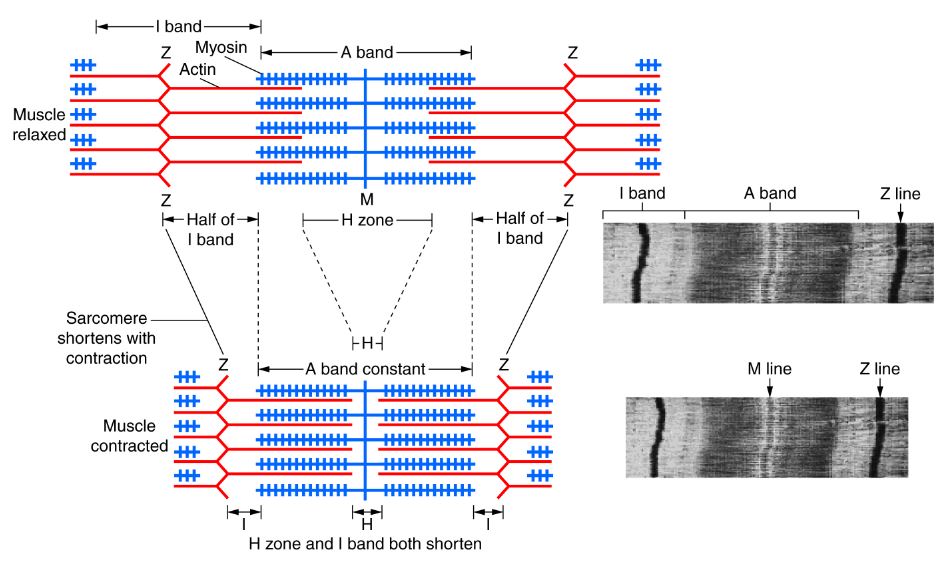
Title: SARCOMERE ANATOMY; Author: courses.candelalearning.com; Site: https://courses.candelalearning.com/olianp/chapter/muscular-levels-of-organization/; License:licensed under the Creative Commons Attribution 4.0 License. Attributions.http://lumenlearning.com/courseware-candela/
Muscle contractures and cramps
Muscle contracture is a term that generally refers to a muscle that has shortened and resists relaxing to its normal resting length. There are many possible causes of a contracture. Some of the reasons that tend to cause long term or even permanent contracture include prolonged immobilization, spasticity, and muscle weakness. Although rare, temporary contractures can occur with severe muscle fatigue resulting in a condition called physiologic contracture. Extreme exercise may lead to a relative depletion of ATP. The lack of ATP prevents the detachment of the myosin head from the actin, preventing the muscle from relaxing. Recall that in order for the myosin head to detach from the actin, ATP must bind to the myosin. Without ATP, the cross bridge remains intact and the muscle becomes rigid.
Though not considered a "contracture" in the normal sense of the word, rigor mortis is muscle rigidity also caused by a depletion of ATP. Rigor mortis is associated with death. It may take hours to fully develop, but cellular death results in the breakdown of intracellular sarcoplasmic reticulum and the leakage of Ca2+ . The Ca2+ then initiates the events that allow cross bridge formation. However, cell death also results in the cessation of ATP production and without ATP to cause the dissociation of myosin heads from actin, the muscle stays in a contracted position and cannot relax or be stretched.
Students often ask us what causes muscle cramps. It appears that there are many things that contribute, and physiologists are still puzzling this out. Below is some information that is mostly FYI that reveals some of the current thinking and theory about muscle cramps:
Another interesting phenomenon of uncontrolled muscle contraction is a cramp. Most people have experienced or will experience a sudden, involuntary, painful contraction at some point. It is very common during or after intense exercise. A characteristic that distinguishes cramps from contractures is that contractures are "electrically silent." This means that we do not see repeated action potentials coming down the motor neurons to the muscle cell. Contractures originate because of a physiological change of the muscle fiber itself and not the motor neuron that innervates it. Cramps, on the other hand are associated with repeated firing of action potentials in the motor neurons. Cramps are found most commonly in muscles of the leg, especially the lower leg and foot. The pathophysiology of cramps is poorly understood. It is suspected that the origin of a cramp may be either central or peripheral. The central theories suggest that there are some changes in the brain or spinal cord that cause an abnormal frequency of action potentials to the lower motor neurons that will innervate skeletal muscle. Peripheral theories suggest that there is some change around peripheral motor neurons that cause them to discharge spontaneously.
Functional use of muscles is a more complicated process than most of us realize. The brain and spinal cord receive afferent (sensory) information that helps us know how and where our body is moving in space. Then, our brain and spinal cord respond by inhibiting some muscle fibers and contracting others. The brain and spinal cord receive a lot of information really fast during intense exercise and action potential patterns are tweaked and enhanced to keep us going and make our movements most successful. The sudden stopping of an intense activity might leave us with action potential delivery mechanisms intended for muscles and movements that are no longer occurring. Perhaps a cramp is a manifestation of the inability of the nervous system to resolve this conflict.
Peripherally, there is significant redistribution of blood flow as the body deals with energy needs and heat buildup. In what is somewhat of a paradox, when a skeletal muscle is contracting blood delivery to the muscle decreases. This is due to the fact that a contracting muscle constricts blood vessels limiting blood flow in that vessel. Over time, during intense exercise, a muscle may contract long and hard enough that blood supply is disrupted in key areas such as the epineurium of motor nerve bundles. Blood flow disruption may be enough to cause slight imbalances in the concentrations of key extracellular ions in localized areas. Exercise also results in water loss through sweat and dehydration and can certainly influence extra cellular ion concentrations as well. There is plenty of anecdotal evidence to suggest that sports drinks balanced with electrolytes can decrease the occurrence of exercise induced cramping. While most athletes will swear that electrolyte enhanced drinks help them, it is difficult to point to an electrolyte imbalance as the ultimate cause of muscle cramps. Maughan (1986), selected a group of marathon runners who experienced cramps during a race and compared their blood tests to those who did not have cramps. There were no significant differences in serum electrolyte levels for the two groups of runners. Maughan's conclusion was that if electrolyte imbalance was the problem it was not likely systemic. Recently, the BBC news outlet reported on a sudden death that occurred in a 23 year old male running a marathon in Hove England. The autopsy reported that sports supplements high in potassium coupled with dehydration contributed to the fatality.
Intense exercise is often the precipitator of a muscle cramp, but exercise is not a necessary requirement to experience a cramp. Many people report cramping at rest or even at night while they are asleep. The theories that suggest that exercise is the cause of cramping are hard to adapt to a cramp that has nothing to do with intense exercise. The mechanisms of cramps at night or at rest are even a bigger puzzle than the ones occurring with exercise. One common theory has been to blame muscle cramps on Ca2+ . People who demonstrate hypocalcemia (low blood Ca2+ levels) generally experience frequent muscle cramps. It has already been illustrated that intracellular Ca2+ is key to muscle contraction, but muscles also depend on the concentration of extracellular Ca2+ to help stabilize the voltage-gated Na + channels. If the extracellular Ca2+ concentration declines then the Na + channels become hypersensitive and have a tendency to "pop" open independent of any outside stimulus. This can lead to uncontrollable cramping. There are several conditions that can cause calcium levels to drop. Thyroid and parathyroid problems, kidney problems, severe dietary deficiencies, pregnancy, and very low vitamin D are a few. It is not likely, however, that generally healthy people will have hypocalcemia during exercise or at rest. The suggestion to drink milk to resolve cramps for most healthy people experiencing them is not likely to be helpful.
We hear all kinds of folklore advice. "Eat a banana, drink milk, drink water, ingest baking soda, etc." Research on the internet on how to stop muscle cramps is like looking up ways to stop the hiccups; everyone has a solution. The take home message on muscle cramps is this:
- There are likely many many different causes and we really don't know much about them or why they occur.
- We need to be very careful to not get caught up in sports drinks, powders, potions, and plans that are sold to us to increase our exercise ability or decrease our pain (like cramps). Remember the guy mentioned above who died doing this (he was only 23!).
- It is pretty normal to get muscle cramps as they are quite common. However, if cramps are getting worse, lasting longer, and coming more often over a period of time, you should seek medical advice as there are several pathologies that have muscle cramps as one of the defining symptoms.
References:
Maughan RJ. Exercise-induced muscle cramp: a prospective biochemical study in marathon runners. J. Sports Med. 1986; spring 4 (1): 31-34.
BBC article: http://www.bbc.com/news/uk-england-sussex-23900695
**You may use the buttons below to go to the next or previous reading in this Module**