SKELETAL MUSCLE: WHOLE MUSCLE PHYSIOLOGY
MOTOR UNITS
Image drawn by BYU-I student Nate Shoemaker Spring 2016
The motor neurons that innervate skeletal muscle fibers are called alpha motor neurons. As the alpha motor neuron enters a muscle, it divides into several branches, each innervating a muscle fiber (note this in the image above). One alpha motor neuron along with all of the muscle fibers it innervates is a motor unit . The size of the motor unit correlates with the function of the muscle. In muscles involved with fine, coordinated control, the motor units are very small with 3-5 muscle fibers per motor neuron. Muscles that control eye movement and muscles in our hands have relatively small motor units. On the other hand in muscles involved with more powerful but less coordinated actions, like the muscles of the legs and back, the motor units are large with 1000s of muscle fibers per motor neuron.
MUSCLE TWITCH
Title: File:1012 Muscle Twitch Myogram.jpg; Author: OpenStax College; Site:https://commons.wikimedia.org/wiki/File:1012_Muscle_Twitch_Myogram.jpg; License: This file is licensed under the Creative Commons Attribution 3.0 Unported license.
When an action potential travels down the motor neuron, it will result in a contraction of all of the muscle fibers associated with that motor neuron. The contraction generated by a single action potential is called a muscle twitch. A single muscle twitch has three components. The latent period, or lag phase, the contraction phase, and the relaxation phase. The latent period is a short delay (1-2 msec) from the time when the action potential reaches the muscle until tension can be observed in the muscle. This is the time required for calcium to diffuse out of the SR, bind to troponin, the movement of tropomyosin off of the active sites, formation of cross bridges, and taking up any slack that may be in the muscle. The contraction phase is when the muscle is generating tension and is associated with cycling of the cross bridges, and the relaxation phase is the time for the muscle to return to its normal length. The length of the twitch varies between different muscle types and could be as short as 10 ms (milliseconds) or as long as 100 ms (more on this later).
If a muscle twitch is just a single quick contraction followed immediately by relaxation, how do we explain the smooth continued movement of our muscles when they contract and move bones through a large range of motion? The answer lies in the ordering of the firing of the motor units. If all of the motor units fired simultaneously the entire muscle would quickly contract and relax, producing a very jerky movement. Instead, when a muscle contracts, motor units fire asynchronously, that is, one contracts and then a fraction of a second later another contracts before the first has time to relax and then another fires and so on. So, instead of a quick, jerky movement the whole muscle contraction is very smooth and controlled. Even when a muscle is at rest, there is random firing of motor units. This random firing is responsible for what is known as muscle tone. So, a muscle is never "completely" relaxed, even when asleep. However, if the neuron to a muscle is cut, there will be no "muscle tone" and this is called flaccid paralysis. There are several benefits of muscle tone: First it takes up the "slack" in the muscle so that when it is asked to contract, it can immediately begin to generate tension and move the limb. If you have ever towed a car you know what happens if you don't take the slack out of the tow rope before starting to pull. The second thing muscle tone does is deter muscle atrophy.
TYPES OF MUSCLE CONTRACTION
Muscle contractions are described based on two variables: force (tension) and length (shortening). When the tension in a muscle increases without a corresponding change in length, the contraction is called an isometric contraction (iso = same, metric=length). Isometric contractions are important in maintaining posture or stabilizing a joint. On the other hand, if the muscle length changes while muscle tension remains relatively constant, then the contraction is called an isotonic contraction (tonic = tension). Furthermore, isotonic contractions can be classified based on how the length changes. If the muscle generates tension and the entire muscle shortens than it is a concentric contraction. An example would be curling a weight from your waist to your shoulder; the bicep muscle used for this motion would undergo a concentric contraction. In contrast, when lowering the weight from the shoulder to the waist the bicep would also be generating force but the muscle would be lengthening, this is an eccentric contraction. Eccentric contractions work to decelerate the movement at the joint. Additionally, eccentric contractions can generate more force than concentric contractions. Think about the large box you take down form the top shelf of your closet. You can lower it under total control using eccentric contractions but when you try to return it to the shelf using concentric contractions you cannot generate enough force to lift it back up. Strength training, involving both concentric and eccentric contractions, appears to increase muscle strength more than just concentric contractions alone. However, eccentric contractions cause more damage (tearing) to the muscle resulting in greater muscle soreness. If you have ever run downhill in a long race and then experienced the soreness in your quadriceps muscles the next day, you know what we are talking about.
Muscle size is determined by the number and size of the myofibrils, which in turn is determined by the amount of myofilament proteins. Thus, resistance training will induce a cascade of events that result in the production of more proteins. Often this is initiated by small, micro-tears in and around the the muscle fibers. If the tearing occurs at the myofibril level the muscle will respond by increasing the amount of proteins, thus strengthening and enlarging the muscle, a phenomenon called hypertrophy. This tearing is thought to account for the muscle soreness we experience after a workout. As mentioned above, the repair of these small tears results in enlargement of the muscle fibers but it also results in an increase in the amount of connective tissue in the muscle. When a person "bulks up" from weight training, a significant percent of the increase in size of the muscle is due to increases in the amount of connective tissue. It should be pointed out that endurance training does not result in a significant increase in muscle size but increases its ability to produce ATP aerobically.
FACTORS THAT INFLUENCE THE FORCE OF MUSCLE CONTRACTION
Obviously our muscles are capable of generating differing levels of force during whole muscle contraction. Some actions require much more force generation than others; think of picking up a pencil compared to picking up a bucket of water. The question becomes, how can different levels of force be generated?
Multiple-motor unit summation or recruitment: It was mentioned earlier that all of the motor units in a muscle usually don't fire at the same time. One way to increase the amount of force generated is to increase the number of motor units that are firing at a given time. We say that more motor units are being recruited. The greater the load we are trying to move the more motor units that are activated. However, even when generating the maximum force possible, we are only able to use about 1/3 of our total motor units at one time. Normally they will fire asynchronously in an effort to generate maximum force and prevent the muscles from becoming fatigued. As fibers begin to fatigue they are replaced by others in order to maintain the force. There are times, however, when under extreme circumstances we are able to recruit even more motor units. You have heard stories of mothers lifting cars off of their children, this may not be totally fiction. Watch the following clip to see how amazing the human body can be. Muscle recruitment. (Video Transcription Available)
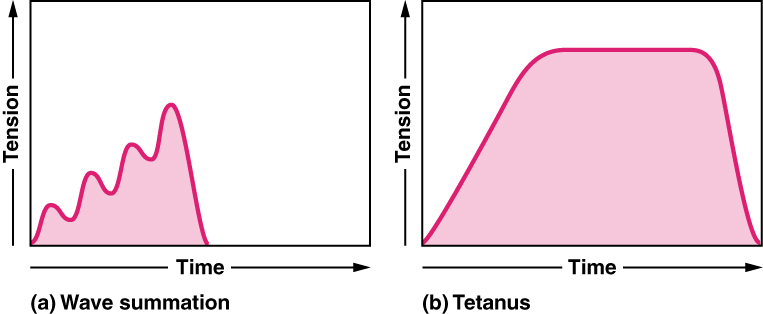
Title: 1013_Summation_Tetanus.jpg; Author: OpenStax; Site: http://cnx.org/contents/14fb4ad7-39a1-4eee-ab6e-3ef2482e3e22@6.6:67/Anatomy-&-Physiology; License: This work is licensed by Rice University under a Creative Commons Attribution License License ( 3.0).
Wave summation: Recall that a muscle twitch can last up to 100 ms and that an action potential lasts only 1-2 ms. Also, with the muscle twitch, there is not refractory period so it can be re-stimulated at any time. If you were to stimulate a single motor unit with progressively higher frequencies of action potentials you would observe a gradual increase in the force generated by that muscle. This phenomenon is called wave summation. Eventually the frequency of action potentials would be so high that there would be no time for the muscle to relax between the successive stimuli and it would remain totally contracted, a condition called tetanus. Essentially, with the high frequency of action potentials there isn't time to remove calcium from the cytosol. Maximal force, then, is generated with maximum recruitment and an action potential frequency sufficient to result in tetanus.
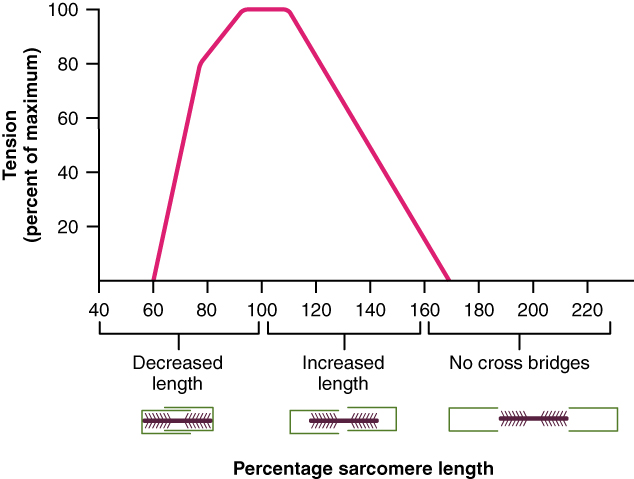
Title: 1011_Muscle_Length_and_Tension.jpg; Author: OpenStax; Site: http://cnx.org/contents/14fb4ad7-39a1-4eee-ab6e-3ef2482e3e22@6.6:67/Anatomy-&-Physiology; License: This work is licensed by Rice University under a Creative Commons Attribution License License ( 3.0).
Initial Sarcomere Length: It has been demonstrated experimentally that the starting length of the sarcomere influences the amount of force the muscle can generate. This observation has to do with the overlap of the thick and thin filaments. If the starting sarcomere length is very short, the thick filaments will already be pushing up against the Z-disc and there is no possibility for further sarcomere shortening, and the muscle will be unable to generate as much force. On the other hand, if the muscle is stretched to the point where myosin heads can no longer contact the actin, then again, less force will be generated. Maximum force is generated when the muscle is stretched to the point that allows every myosin head to contact the actin and the sarcomere has the maximum distance to shorten. In other words, the thick filaments are at the very ends of the thin filaments. These data were generated experimentally using frog muscles that were dissected out and stretched between two rods. Intact muscles in our bodies are not normally stretched very far beyond their optimal length due to the arrangement of muscle attachments and joints.
However, you can do a little experiment that will help you see how force is lost when a muscle is in a very short or a very stretched position. This experiment will use the muscles that help you pinch the pad of your thumb to the pads of your fingers. These muscles are near maximal stretch when you extend your arm and also extend your wrist. As your wrist is cocked back into maximal extension, try to pinch your thumb to your fingers. See how weak it feels? Now, gradually flex your wrist back to a straight or neutral position. You should feel your pinch get stronger. Now, flex your elbow and your wrist. With your wrist in maximal flexion, the muscles you use to pinch with are near their most shortened position. Try pinching again. It should feel weak. But, again, as you extend your wrist back to neutral you should feel your pinch get stronger.
ENERGY SOURCE FOR MUSCLE CONTRACTION
The ultimate source of energy for muscle contraction is ATP. Recall that each cycle of a myosin head requires an ATP molecule. Multiply that by all of the myosin heads in a muscle and the number of cycles each head completes each twitch and you can start to see how much ATP is needed for muscle function. It is estimated that we burn approximately our entire body weight in ATP each day so it becomes apparent that we need to constantly replenish this important energy source. For muscle contraction, there are four ways that our muscles get the ATP required for contraction.
- Cytosolic ATP: This ATP represents the "floating" pool of ATP, or that which is present and available in the cytoplasm. This ATP requires no oxygen (anaerobic) to make it (because it is already there) and is immediately available but it is short lived. It provides enough energy for a few seconds of maximal activity in the muscle-not the best source for long term contraction. Nevertheless, for the muscles of the eyes that are constantly contracting quickly but for short periods of time, this is a great source.
- Creatine Phosphate: Once the cytosolic stores of ATP are depleted, the cell calls upon another rapid energy source, Creatine Phosphate. Creatine phosphate is a high energy compound that can rapidly transfer its phosphate to a molecule of ADP to quickly replenish ATP without the use of oxygen. This transfer requires the enzyme creatine kinase, an enzyme that is located on the M-line of the sarcomere. Creatine phosphate can replenish the ATP pool several times, enough to extend muscle contraction up to about 10 seconds. Creatine Phosphate is the most widely used supplement by weight lifters. Although some benefits have been demonstrated, most are very small and limited to highly selective activities.
- Glycolysis: Glycolysis, as the name implies, is the breakdown of glucose. The primary source of glucose for this process is from glycogen that is stored in the muscle. Glycolysis can function in the absence of oxygen and as such, is the major source of ATP production during anaerobic activity. This series of chemical reactions will be a major focus in the next unit. Although glycolysis is very quick and can supply energy for intensive muscular activity, it can only be sustained for about a minute before the muscles begin to fatigue.
- Aerobic or Oxidative Respiration: The mechanisms listed above can supply ATP for maybe a little over a minute before fatigue sets in. Obviously, we engage in muscle activity that lasts much longer than a minute (things like walking or jogging or riding a bicycle). These activities require a constant supply of ATP. When continuous supplies of ATP are required, the cells employ metabolic mechanisms housed in the mitochondria that utilize oxygen. We normally refer to these processes as aerobic metabolism or oxidative metabolism. Using these aerobic processes, the mitochondria can supply sufficient ATP to power the muscle cells for hours. The down side of aerobic metabolism is that it is slower than anaerobic mechanisms and is not fast enough for intense activity. However, for moderate levels of activity, it works great. Although glucose can also be utilized in aerobic metabolism, the nutrient of choice is fatty acids. As described below, slow-twitch and fast-twitch oxidative fibers are capable of utilizing aerobic metabolism
FATIGUE
When we think of skeletal muscles getting tired, we often use the word fatigue, however, the physiological causes of fatigue vary considerably. At the simplest level, fatigue is used to describe a condition in which the muscle is no longer able to contract optimally. To make discussion easier, we will divide fatigue into two broad categories: Central fatigue and peripheral fatigue. Central fatigue describes the uncomfortable feelings that come from being tired, it is often called "psychological fatigue." It has been suggested that central fatigue arises from factors released by the muscle during exercise that signal the brain to "feel" tired. Psychological fatigue precedes peripheral fatigue and occurs well before the muscle fiber can no longer contract. One of the outcomes of training is to learn how to overcome psychological fatigue. As we train we learn that those feelings are not so bad and that we can continue to perform even when it feels uncomfortable. For this reason, elite athletes hire trainers that push them and force them to move past the psychological fatigue.
Peripheral fatigue can occur anywhere between the neuromuscular junction and the contractile elements of the muscle. It can be divided into two subcategories, low frequency (marathon running) and high frequency (circuit training) fatigue. High frequency fatigue results from impaired membrane excitability as a result of imbalances of ions. Potential causes are inadequate functioning of the Na+/K+ pump, subsequent inactivation of Na+ channels and impairment of Ca2+ channels. Muscles can recover quickly, usually within 30 minutes or less, following high frequency fatigue. Low frequency fatigue is correlated with impaired Ca2+ release, probably due to excitation coupling contraction problems. It is much more difficult to recover from low frequency fatigue, taking from 24 hours to 72 hours.
In addition, there are many other potential fatigue contributors, these include: accumulation of inorganic phosphates, hydrogen ion accumulation and subsequent pH change, glycogen depletion, and imbalances in K+. Please note that factors that are not on the list are ATP and lactic acid, both of which do not contribute to fatigue. The reality is we still don't know exactly what causes fatigue and much research is currently devoted to this topic.
SKELETAL MUSCLE FIBER TYPES
Classically, skeletal muscle fibers can be categorized according to their speed of contraction and their resistance to fatigue. These classifications are in the process of being revised, but the basic types include:
- Slow twitch oxidative (type I) muscle fibers,
- Fast-twitch oxidative-glycolytic (Type IIA) muscle fibers, and
- Fast-twitch glycolytic (Type IIX) fibers.
Fast-twitch (type II) fibers develop tension two to three times faster than slow-twitch (type I) fibers. How fast a fiber can contract is related to how long it takes for completion of the cross-bridge cycle. This variability is due to different varieties of myosin molecules and how quickly they can hydrolyze ATP. Recall that it is the myosin head that splits ATP. Fast-twitch fibers have a more rapid ATPase (splitting of ATP into ADP + Pi) ability. Fast-twitch fibers also pump Ca2+ ions back into the sarcoplasmic reticulum very quickly, so these cells have much faster twitches than the slower variety. Thus, fast-twitch fibers can complete multiple contractions much more rapidly than slow-twitch fibers. For a complete list of how muscle fibers differ in their ability to resist fatigue see the table below:
Slow Twitch Oxidative (Type I) | Fast-twitch Oxidative (Type IIA) | Fast-Twitch Glycolytic (Type IIX) | |
Myosin ATPase activity | slow | fast | fast |
Size (diameter) | small | medium | large |
Duration of contraction | long | short | short |
SERCA pump activity | slow | fast | fast |
Fatigue | resistant | resistant | easily fatigued |
Energy utilization | aerobic/oxidative | both | anerobic/glycolytic |
capillary density | high | medium | low |
mitochondria | high numbers | medium numbers | low numbers |
Color | red (contain myoglobin) | red (contain myoglobin) | white (no myoglobin) |
In human skeletal muscles, the ratio of the various fiber types differs from muscle to muscle. For example the gastrocnemius muscle of the calf contains about half slow and half fast type fibers, while the deeper calf muscle, the soleus, is predominantly slow twitch. On the other hand the eye muscles are predominantly fast twitch. As a result, the gastrocnemius muscle is used in sprinting while the soleus muscle is important for standing. In addition, women seem to have a higher ratio of slow twitch to fast twitch compared to men. The "preferred" fiber type for sprinting athletes is the fast-twitch glycolytic, which is very fast, however, most humans have a very low percentage of these fibers, < 1%. Muscle biopsies of one world class sprinter revealed 72% fast twitch fibers and amazingly 20% were type IIX. The Holy Grail of muscle research is to determine how to change skeletal muscle fibers from one type to another. It appears that muscle fiber types are determined embryologically by the type of neuron that innervates the muscle fiber. The default muscle appears to be slow, type I fibers. If a muscle is innervated by a small neuron that muscle fiber will remain slow, whereas large mylenated fibers induce the fast isoforms. In addition, the frequency of firing rates of the neuron also alters the muscle fiber type. Research suggests that humans have subtypes of fibers, making up about <5% of the muscle, that are dually innervated and allow for switching between slow and fast to occur. Generally, it would appear that genetics determine the type of innervation that occurs and subsequent muscle fiber types and that training may be able to slightly alter the ratios due to the dually innervated muscles. However, since <5% have dual innervation, genetics is going to play a much greater role in your fiber types than your training.
**You may use the buttons below to go to the next or previous reading in this Module**