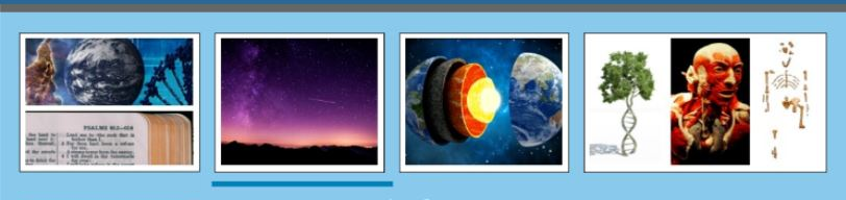
Explore 2.10 How Planetary System Order Emerges
Learning Objectives
By the time you have completed the 2.10. Introduction & Exploration Activities, you should be able to:
- Understand the meaning of accretion and illustrate how this process builds planetary bodies.
- Describe the nature and origin of these types of order in the solar system:
- Matter is heavily concentrated in the sun, planets, and planetary bodies—which are separated by vast ‘emptiness'
- The materials that comprise planetesimals and planets in our solar system are distributed radially through the solar system: planetary bodies that formed near the Sun are composed primarily of rock & metal, whereas those that formed farther from the Sun are enriched in ices, gasses, and organic compounds relative to relative to those that formed near the Sun
- Planetary bodies in our solar system orbit the Sun and spin about their axes in about the same direction, and they share the same orbital plane
- None of the ages of the oldest samples from planetary bodies in our solar system are young; instead, they all formed early in the history of the solar system, clustering ~4.5 billion years ago
- Understand the types of materials (metal, rock, ice, gas) that accrete to form planets and other planetary bodies such as asteroids, comets, and moons. Also, describe how our planetary/solar system formed from a nebula in terms of lawful self-assembly—using terms such as nebula, orbit, gravity, temperature, gas, dust, rock, metal, ice, accretion, proplyd, planet, planetesimals, planetary body, initial state, transition, and final state.
Accretion
As solids orbit within the proplyd, gentle bumps can cause solid particles to stick together, and additional encounters can cause these to grow—like the dust bunnies that form under your bed. Through encounters, these agglomerations of dust continue growing—as shown in the simulation below (20 sec), into solid pebble-sized particles. Further collisions cause the pebbles to grow to fist-sized and then boulder-sized rocks, eventually becoming ‘planetesimals’—tiny planets. As planetesimals grow they become protoplanets, which finally form planets. This process—in which planets form by the building of ever-larger solid bodies—is called accretion.
Order in the Solar System
We began learning about the creation of our planetary system when we learned about the creation of the Sun. Recall from that discussion how material within globules in the nebula begin to collapse where gravitational effects become strong enough to overcome gas pressure in the nebula. Thus, globules form in cooler regions that compress more easily or in regions where external galactic process produce greater compression. Inside a collapsing globule, gravity pulls material towards the center where a new star will form.
During collapse, density differences and random gas motions originally present in the cloud interact, causing the globule to spin in a particular direction. Like a skater pulling in their arms and legs, as the globule shrinks it spins faster. This rotation causes the blob-shaped globule to flatten out—much like forming a flat pizza out of a ball of dough by spinning it overhead. The flattened disk, which surrounds the central star-forming region, consists of gases and minor dust—the same materials that form the central star. The image below-left (which was drawn by one of your colleagues, a BYU-Idaho student), shows a protoplanetary disk, or proplyd, in which planets and a star(s) will form. The image below-right is a collage of a few of the proplyds that humanity has photographed in the Orion Nebula. If you’ve ever wondered why planetary systems are ‘flat’, now you know! The process of forming a proplyd by globule collapse from a nebula takes about 100,000 years—by which time hydrogen fusion has begun in the core of the protostar and is experiencing the ever-dampening interactions that will eventually produce the stable balance between gravity and gas-pressure that characterizes normal stars.
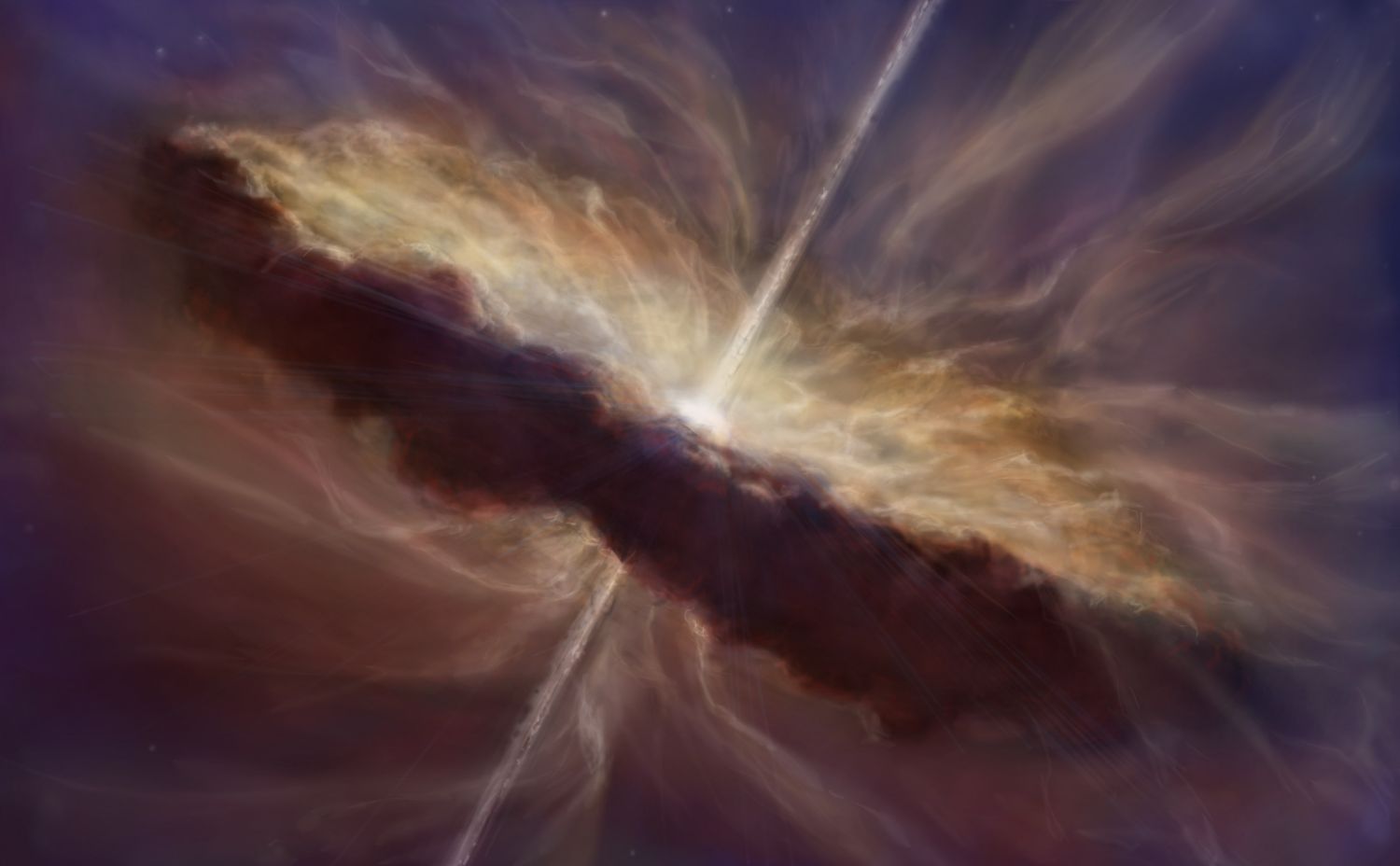
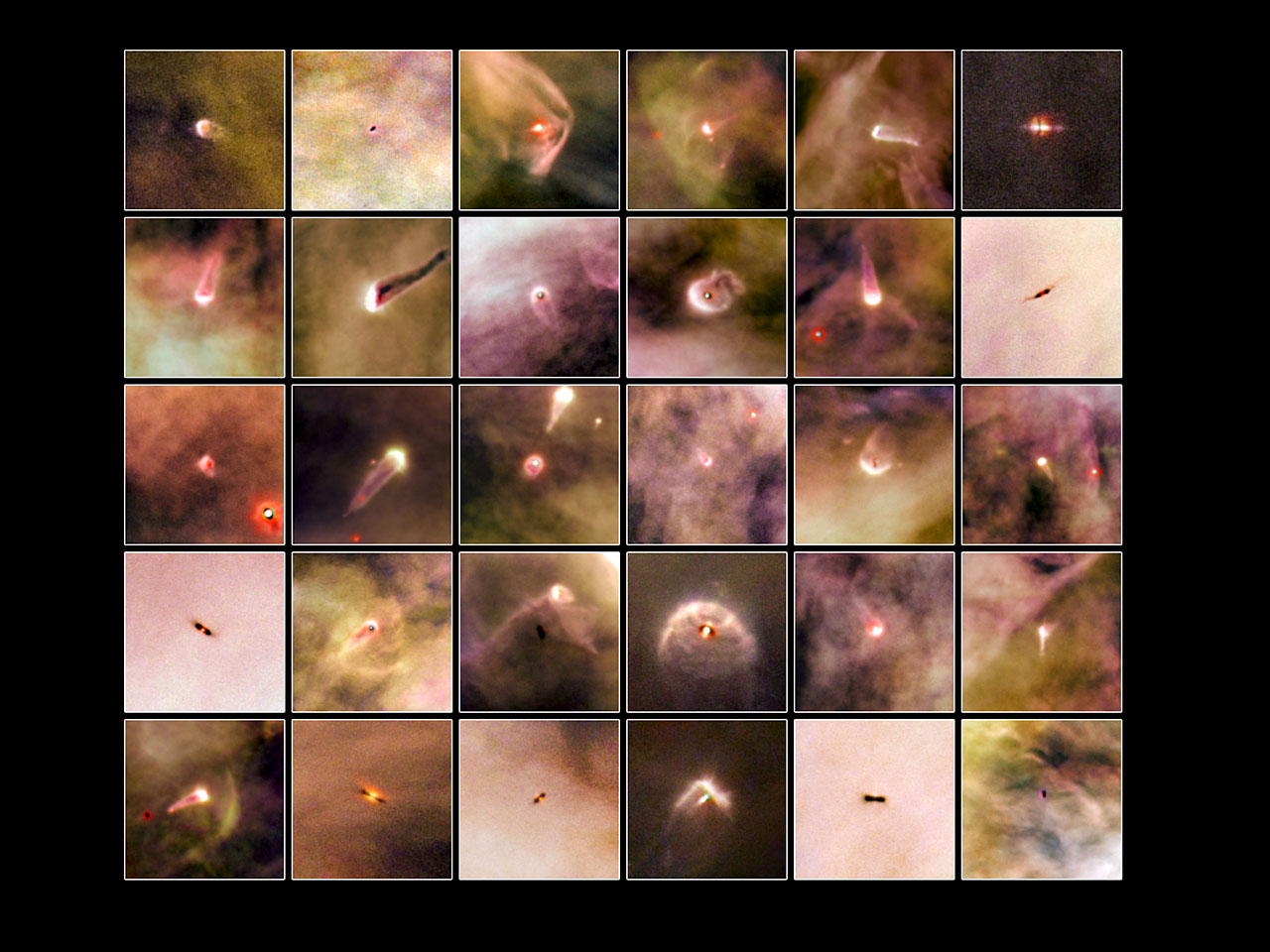
We provide the questions below to help you deepen your understanding of proplyds and to allow you to assess the relationship between the attributes of proplyds and the characteristics of our solar system, which was produced from a proplyd.
Notice, in the image above that the proplyd consists of a central star-forming region surrounded by a relatively uniform disk of gas and dust. Compare the distribution of matter in the proplyd to that in the nebula that produced the proplyd and to that in our solar system. Why did the diffuse distribution of matter in the nebula become more concentrated in the proplyd (in the central dense region and in the surrounding disk) and then further concentrated in our Solar System (in the Sun, planets, and other planetary bodies such as asteroids and comets)?
Describe the direction in which the gasses and dust inside the disk are moving.
Using what you know about the relationship between gravity and rotational motion (orbit), describe why the material in the proplyd doesn’t fall directly into the center and become part of the newly forming star.
Notice the tendrils of matter above and below the plane of the proplyd. These tendrils lead from the surrounding nebula to the proplyd. Describe what is happening to the materials that comprise the tendrils.
The material inside the tendrils is accelerated as it moves toward proplyd. Thus, it is moving rapidly when it encounters the disk. In contrast, the material inside the disk rotates relatively slowly. Predict how the rapidly moving material entering the disk from the tendrils affects the disk. Record your prediction in the box below.
Interactions between elements (gas & dust) in the disk tend to slow their orbits about the central star. Matter that slows down moves towards the center of the proplyd. Thus, in addition to rotating around the star, some matter flows towards the star. Also, the newly formed star lies at the center of the disk. How does in-falling gas (& dust) and the location of the new star relate to the distribution of rock, metal, ice, and gas in the early solar system?
What does the vaporizing of pre-existing solids and subsequent cooling of the disk imply for the ages of the ages of the oldest solid materials in the solar system?
How does the shared rotational motion of matter in the protoplanetary disk relate to the orbital directions and spins of the planets that form in the proplyd?
How does the flat character of the protoplanetary disk relate to the orbital planes of the planets that form in the proplyd?
Formation of Planets, Planetary Bodies, and our Solar System
Forming Planetary Systems
System Boundary
Elements
Source of Energy
Initial state
Governing Process(es)
Push(es)
Response(s)
Transition begins with
Transition ends with
Final state
Governing Process(es)
Push(es)
Response(s)
To deepen your understanding of the processes involved in forming planets and planetary systems, watch the video below (3 min 20 sec).
